Mitochondria are the body’s power plants, fuelling our cells. New research shows they play a role in many aspects of keeping us healthy – and could be the key to unlocking treatments for chronic diseases, including Parkinson’s.
At Cambridge University’s MRC Mitochondrial Biology Unit, Michal Minczuk is one of a growing number of scientists around the world aiming to find new ways of improving mitochondrial health. This line of research could help provide much-needed treatments for people with long Covid, as well as revolutionising our understanding of everything from neurodegenerative illnesses such as Parkinson’s disease to the ageing process.
Mitochondria, tiny tube-shaped structures that are found in their hundreds, sometimes thousands, in nearly all of our cells, are best known as the body’s power plants, continuously converting the food we eat into ATP, a complex chemical that acts as a form of energy currency for cells. Without ATP, every one of our cells, from the brain to the muscles, would lack the fuel they need to keep churning away, and our organs would swiftly grind to a halt.
“One of the complexities of mitochondria is that they have their own DNA, separate from the DNA stored in the nuclei of our cells.”
But while mitochondria are often typecast as energy factories, scientists have repeatedly discovered that they do far more than simply generate ATP. For one thing, they can help keep us warm when we are cold via an alternative form of heat generation to shivering, and studies have suggested that mitochondria in the eye even play a role in focusing light on to the retina, helping us perceive our environment.
In fact, the more we look, the more we find that they contribute to the many building blocks of life that keep us healthy, from synthesising the protein haemoglobin, which transports oxygen in the bloodstream, to storing calcium, and even the immune system response. While mitochondria sustain our cells, they also play a critical role in the natural process of cell death that occurs over and over again throughout our lives, identifying old and damaged cells which must be cleared away and destroyed.
Put simply they are vital to our survival, but like much of the body’s innate machinery, we only notice them when they start to go wrong. “Mitochondria are involved in many processes so when they don’t function well, this can precipitate different types of dysfunction in the human body leading to disease,” says Minczuk.
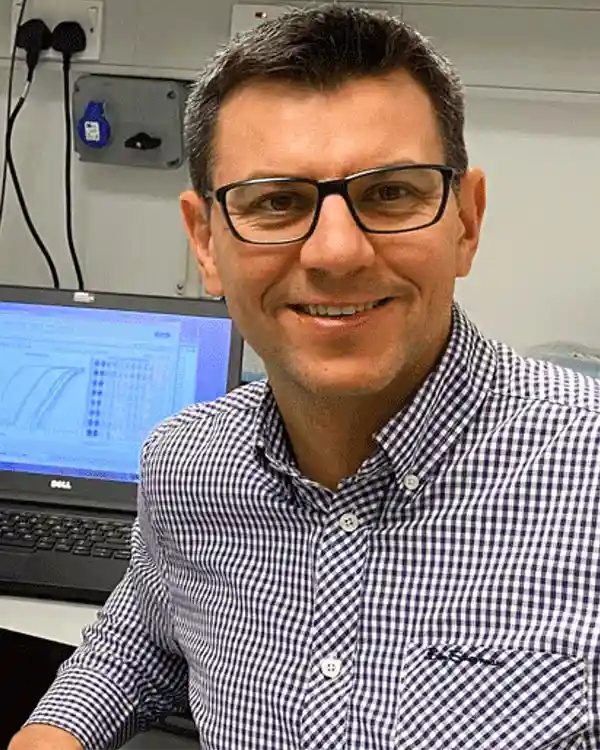
One of the unique complexities of mitochondria is that they have their own DNA, separate from the DNA stored in the nuclei of our cells, which comes from both parents. Mitochondrial DNA (mtDNA) is passed down from the mother only, and consists of fewer than 17,000 base pairs, compared with 3.3bn in the nucleus. But it still encodes specific instructions for a number of proteins, and over the past decade, scientists have found that mutations in mtDNA that prevent mitochondria from functioning normally can affect our health, contributing to a variety of chronic illnesses.
The most drastic cases are so-called mitochondrial diseases where mutations in mtDNA are acquired genetically. They affect around one in 4,300 people, and the consequences are grave. The life expectancy for most patients is between 10 and 35 years, with most dying from general body wasting owing to brain or muscle damage, or impairments to organs such as the heart and kidneys. But studies have also shown that mutations can accumulate in mtDNA as we age, and Minczuk’s research group at the University of Cambridge MRC mitochondrial biology unit is particularly interested in the role this might play in Parkinson’s.
It is thought that some Parkinson’s patients have genetic mutations that prevent damaged mitochondria being eliminated and replaced with healthy versions – a process called autophagy. As a result, the existing mitochondria in the body accrue more and more mutations, with damaging consequences for cells such as neurons, which rely heavily on the energy they supply.
But the rise of new gene-editing techniques may offer new treatment solutions in the years to come, initially for mitochondrial diseases but possibly for other illnesses too. This has been a challenge because Crispr technology – which uses a piece of RNA to guide an enzyme to a specific DNA location where it cuts out a mutation – cannot be used to tweak mitochondria, as it is not possible to deliver RNA into mtDNA.
However over the past few years, scientists including Minczuk have designed enzymes that can achieve the same effect as Crispr without requiring RNA. While studies are still being conducted on rodents, this offers enormous future potential.
“We’re slowly gathering the tools to be able to modify the mitochondrial genome in animal cells,” Minczuk says. “Right now we could eliminate existing mutations, changing the genetic make-up of mitochondria, but we also want to be able to trigger new mutations. This would allow us to study Parkinson’s in far more detail. We could take a healthy mouse, for example, and introduce mutations seen in Parkinson’s patients, and see what happens. Would that trigger the onset of symptoms?”
Treating Long Covid
While hacking the mitochondrial genome could change healthcare in years to come, finding more immediate ways of improving mitochondrial health could help the millions of people with long Covid and chronic fatigue syndrome, also known as ME/CFS.
At Oxford University, cardiologist Betty Raman is currently in the middle of running a clinical trial to see whether an amino acid cocktail known as AXA1125, produced by Massachusetts-based biotech Axcella Therapeutics, can help long Covid patients where fatigue is by far the dominant symptom.
“The drug is a powdered drink, consumed three times a day along with meals, and we’re hoping that it will help people with their energy levels and fatigue,” she says. “The idea is that it can give the mitochondria additional fuel to produce energy, and help repair damaged mitochondria. Hopefully, by the end of July, we should have some top line results to report.”
The idea that mitochondria may be involved in the ailments of some of those with long Covid arises from research conducted by Raman and others on patients who find themselves chronically exhausted by exercise following Covid-19, despite showing no obvious heart or lung abnormalities. This symptom is often referred to as post-exertional malaise (PEM), and is also experienced by people with genetic mitochondrial diseases.
In long Covid patients with PEM, Raman has found that their muscles struggle to extract oxygen from the blood as efficiently as might be expected. After coming across research that showed that mitochondria in white blood cells were not as efficient in generating ATP in patients recovering from Covid-19, she concluded that this might be the root cause.
But why do the mitochondria of these patients become sluggish in generating ATP? David Systrom, a pulmonary and critical care doctor at Brigham & Women’s Hospital, Boston, believes he has found answers through studying patients with ME/CFS, an illness that in many cases is precipitated by viral infections such as Epstein-Barr and bears many similarities to long Covid.
When Systrom studied the mitochondrial DNA of these patients it appeared to be normal, but after taking a deep look and conducting muscle biopsies, he identified abnormalities at the electron level, deep within the mitochondria.
“In both ME/CFS and long Covid it’s most likely that these are acquired forms of mitochondrial dysfunction, perhaps related to the initial infection itself or an autoimmune response to a virus or both,” Systrom says. “This impedes the mitochondrial machinery, but doesn’t affect the DNA itself, and it means the mitochondria then fail to generate appropriate amounts of ATP to serve the needs of the muscles.”
Systrom is now running his own clinical trial in both ME/CFS and long Covid patients, in partnership with Japanese drug company Astellas, which has developed a drug that aims to restore normal mitochondrial metabolism.
Both Raman and Systrom agree that mitochondrial dysfunction is only likely to be a factor in a subset of long Covid and ME/CFS patients. However, because mitochondria are so ubiquitous throughout the body, damage inflicted to these structures across different organ types could contribute to the wide range of different symptoms that patients tend to report.
A common ailment reported by people with long Covid and ME/CFS is dysautonomia, a peculiar condition that causes a rapid increase in heartbeat and lightheadedness when patients attempt any form of activity. Raman says that this is often caused by damage to small sensory nerves in the skin, something that has been associated with mitochondrial dysfunction.
“There is a theory that the mitochondrial problem may come first,” she says. “And because nerves are high energy tissues, they are particularly dependent on normal mitochondrial function and ATP production.”
Learning From Elite Athletes
Different cell types have different numbers of mitochondria, owing to the varying energy requirements from one organ to the next. Organs with particularly high energy demands such as the brain, the heart and the pancreas tend to have more, which is why dysfunctional mitochondria have been linked to everything from cancer to type 2 diabetes and cardiovascular problems.
While mitochondria are not the main driving factor in any of these diseases, they are thought to be a key secondary factor. “The majority of heart failure or cardiac dysfunction is believed to be mediated by mitochondrial dysfunction involving the heart,” says Raman. “There’s a big metabolic component, and it has to do with the fact that the heart relies very much on continuous oxygen supply, but also that mitochondria are sensitive structures and can be affected by a number of risk factors.”
“When you get down to the cellular level, there are some surprising overlaps between exercise and disease, at least in the muscles.” — Chris Perry
As a result, if mitochondrial drugs prove effective in long Covid and ME/CFS, they may have applications in other illnesses, while mitochondrial DNA editing to understand the effects of various mutations could shed further light on how the ageing process manifests in our cells.
Scientists are also taking some more left-field approaches to finding ways to improve mitochondrial health. At York University in Toronto, Chris Perry is looking at what we can learn from the mitochondria of elite athletes to help those with muscle diseases and even age-related sarcopenia.
As an example, Perry points out that endurance runners have high numbers of extra-efficient mitochondria which fuse to form extensive networks throughout their muscle tissues to deal with the stresses of prolonged exercise. Understanding the pathways that trigger mitochondria to adapt in this manner could lead to therapeutics to help people with different diseases, or keep us healthier in old age. This is already taking place in clinical trials, which have found that the dietary supplement urolithin A seems to improve mitochondrial health in older adults.
“When you get down to the cellular level, there are some surprising overlaps between exercise and disease, at least in the muscles,” says Perry. “Exercise creates enormous cellular stressors. It depletes ATP reserves, it causes physical strain on the cell membranes in the cytoskeleton, and it acidifies the muscle cells, which is exactly what happens in certain diseases.”
Studies have also shown that exercise itself can improve mitochondrial health in older adults who lead sedentary lifestyles, triggering proteins in mitochondria to cluster together in ways that allow them to pass electrons more efficiently.
“The basis of life is adaptation,” says Perry. “And that’s why exercise is good for us, because it exposes our cells to different stressors, which triggers these dedicated cellular feedback pathways to kick into action and regulate the situation. So when we exercise again, it is handled more efficiently. You slowly build those capacities as a result of that stress.”